Ethanol Production from Coconut Husk Biomass
By Dr. Luíza Favaratto
Global scenario on climate change
Modern transportation, with approximately 1.47 billion vehicles worldwide, propels significant energy demand. Unfortunately, the transport sector alone is responsible for 20% of global carbon dioxide (CO2) emissions, exacerbating pollution levels. What’s more concerning is that transportation is the fastest-growing energy-consuming sector, expanding at an average rate of 2% per year. Given Canada’s vast size, low population density, dispersed communities, reliance on transportation for remote industries, it is unlikely that transportation will be reducing. Therefore, other means to reduce the emissions of transportation is necessary.
One of the most common policies aimed at promoting renewable fuels in transport is the blending mandates for biofuels. These mandates require a certain percentage of renewable fuel to be mixed with traditional transportation fuel. However, with a growing population, the number of vehicles entering the global market annually is projected to triple by 2050, from 1 to 3 billion. There is a lot of attention paid to the capability of ethanol to reduce greenhouse gas emissions (GHG). But it also plays an important role in reducing harmful tailpipe pollution, such as carbon monoxide, hydrocarbons, air toxics like benzene, and fine particulate matter.
In line with the global goal of achieving net-zero emissions by 2050, various policies have been put in place, including Fuel Economy Standards, the Paris Agreement (2015), Renewable Fuel Standards (RFS), and Clean Fuel Regulations (CFR), among others. The RFS mandates increasing amounts of renewable fuel to be blended into transportation fuel each year, reaching 36 billion gallons by 2022. Whereas CFR aims to reduce up to 26 million tonnes (Mt) of GHG emissions by 2030. Such a reduction is equivalent to removing two weeks’ worth of GHG emissions from the Canadian economy.
Second-generation ethanol
Second-generation (2G) ethanol, or bioethanol, is a more sustainable alternative to traditional fossil fuels. Unlike first-generation ethanol, which relies on sugars or grains, 2G ethanol uses lignocellulosic material like agricultural and forestry waste. Lignocellulosic material comes from plants like woods and often agricultural byproducts, therefore as an ethanol source is not in direct competition with food production. It is usually composed of a high lignin content, cellulose, and hemicellulose. While cellulose provides the main structural support and hemicellulose helps with flexibility, lignin fills in the gaps and adds resistance to decay and damage. Together, they create a strong, protective barrier around plant cells, enabling it to stand tall and withstand environmental challenges.
Using lignocellulosic biomass not only circumvents the issue of ethanol-food competition but also repurposes non-edible segments of plants. The reduction in GHG emissions achieved by 2G ethanol compared to gasoline can go up to 52%, contingent upon the feedstock utilized. This makes it a crucial player in curbing air pollution.
Furthermore, when choosing biomass sources, it’s essential to consider the prevalent residues produced in particular regions. For instance, in the United States, corn is extensively utilized for first-generation ethanol production, resulting in significant corn stover waste generation. Consequently, the US has begun producing second-generation ethanol derived from corn stover to address this surplus. Conversely, Brazil, being a major sugarcane producer, generates large quantities of sugarcane bagasse waste. Hence, Brazil has initiated second-generation ethanol production from this abundant byproduct. Canada on the other hand, started producing 2G ethanol from mixed municipal solid waste.
Green coconut as biomass
Coconuts, beloved for their taste and economic value, have significant environmental implications, especially in top-producing countries like Indonesia, the Philippines, India, Brazil, and Sri Lanka. In 2022, a massive 62.4 million tons of green coconuts were produced globally.
Improper disposal leads to environmental issues as they often end up in inappropriate locations. Besides, as green coconuts decompose naturally, they release methane (CH4), a potent GHG that exacerbates climate change. This methane can infiltrate the soil and pollute groundwater, contributing to the production of landfill leachate.
The green coconut consists of epicarp, mesocarp (husk), endocarp, solid albumen (coconut meat), and liquid albumen (coconut water) (Figure 1). However, only the solid and liquid albumen are consumed, leaving the remaining 80-85% of the coconut as natural waste.
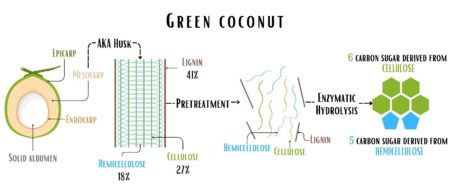
Source: Information gathered from Bolivar-Telleria et al. (2018); Sangian and Widjaja (2018); Li et al. (2022); and Gbenebor et al. (2023).
Green coconut ethanol production
In the realm of 2G ethanol production, challenges arise around unlocking sugars trapped within lignin, a process known as pretreatment (Figure 1). This step involves removing tough substances like lignin, making it easier to access the sugar (cellulose and hemicellulose) and break them down into simple sugars. One common method of pretreatment is alkaline pretreatment. Subsequently, enzymes catalyze enzymatic hydrolysis, breaking down large carbon chain sugars (cellulose and hemicellulose) into smaller carbon chain sugars (e.g. glucose and xylose, respectively). This eco-friendly process operates under mild conditions, although efforts to reduce enzyme production costs and improve efficiency persist.
Fermentation
Following pretreatment and enzymatic hydrolysis, the fermentation process, depicted in Figure 2, completes the ethanol production journey. Efficient ethanol output is crucial, necessitating the use of robust microorganisms capable of enduring inhibitory substances and utilizing the various types of sugars present in lignocellulosic material. These microorganisms convert sugars into ethanol during fermentation.
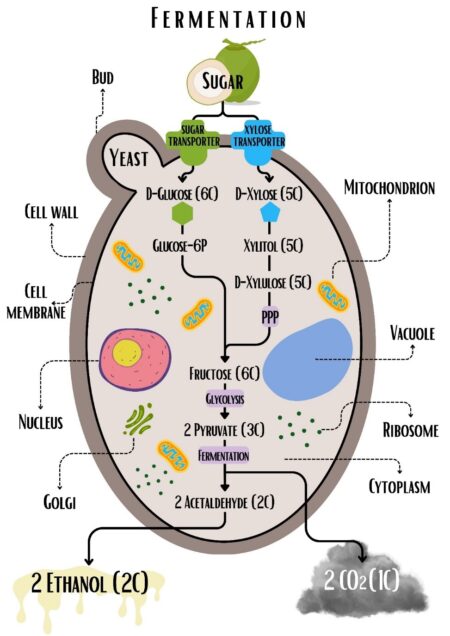
Source: Information gathered from Feldmann (2011); Favarato (2019); Selim (2020); and Perez (2022).
Usually, this process is carried out by yeast (unicellular fungus), which is synonymous with Saccharomyces cerevisiae – a name given to a yeast discovered in malt in 1837 – in connection with brewing beer. This notion immediately recalls that yeast is probably the oldest domesticated organism – it was used for brewing as early as Sumer and Babylon around 6000 BC. In parallel, strains of S. cerevisiae were employed in the production of wine in Georgia and for the fermentation of dough in ancient Egypt. This microorganism has been used for centuries because it is physiologically adapted to the multiple stress conditions imposed during the fermentation process and is used in the fermentation industry to produce cachaça, wine, beer, bread, and ethanol with high production yield.
While it’s true that ethanol production generates CO2 as a byproduct of fermentation, the overall GHG emissions associated with ethanol are lower compared to those from fossil fuels. This is because biomass removes CO2 from the atmosphere throughout its lifetime via photosynthesis, creating a closed carbon cycle. Besides, biogenic CO2 from ethanol biorefineries is a valuable co-product, used in diverse applications like beverage carbonation and wastewater treatment. Actually, ethanol producers are now exploring new opportunities for captured CO2, including geological sequestration and its integration into various industrial products.
GM Yeast
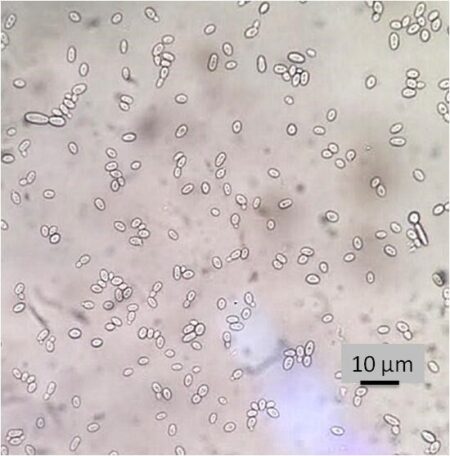
Besides the production of hexoses (i.e. glucose) from green coconut husk, this biomass also produces pentoses such as xylose (Figure 1). High production, then, is synonymous with using all the sugars available in the process. The great majority of S. cerevisiae strains unfortunately are not able to ferment pentoses. The scientists then started developing Genetically Modified (GM) yeasts (optical microscopy of a GM yeast on the right) that are capable of fermenting pentoses and hexoses, increasing the yield of the process.
Indeed, possessing a S. cerevisiae strain proficient in utilizing both hexoses and pentoses presents a significant advantage. However, this characteristic alone may not suffice to ensure the commercial viability of ethanol production. High Hydrostatic Pressure (HHP) has been employed as a method to simulate the stresses encountered by S. cerevisiae during fermentation, including thermal, osmotic, and ethylic stresses. In the study developed by Favarato (2019), HHP was applied for 130 generations of the G2-104 strain, a 2G ethanol strain capable of fermenting both glucose and xylose, utilizing green coconut husk as the carbon source under HHP of 50 MPa. This approach aimed to enhance the fermentation process and improve the efficiency of 2G ethanol production from green coconut husk. Consequently, a notable 90% increase in fermentative capacity was observed as a result of this treatment.
Coconut paving the green road to reducing transport GHGs
Green coconut husk biomass presents a promising avenue for addressing environmental degradation and economic constraints. Through advanced second-generation ethanol production techniques, such as leveraging lignocellulosic components and employing genetically modified yeast strains capable of fermenting both hexoses and pentoses, we can transform this waste into a valuable resource for sustainable energy. While challenges like pentose fermentation and process viability exist, innovations like HHP treatment offer more solutions to enhance ethanol yield.
The potential of cellulosic ethanol in reducing GHG emissions by up to 86% underscores the importance of continued research and collaboration to scale up these technologies. Ethanol’s integration into gasoline has already demonstrated significant environmental benefits, the equivalent of removing 12 million cars from the road for an entire year in 2023, aligning with global emissions policies. By embracing renewable energy sources and innovative approaches to waste biomass utilization, such as green coconut husks, we can simultaneously combat climate change and foster social and economic progress, driving us toward a greener future.
About Me
Hello there! I’m Dr. Luíza Favaratto, a researcher at the Laboratory of Biotechnology Applied to Agribusiness (UFES – Brazil). In my journey through science, I’ve specialized in genetically edited and modified organisms, mastering biosafety protocols and legislation. I’ve also led groundbreaking projects in waste reuse, like 2G ethanol production, and explored life in extreme environments, such as the deep sea. I’m dedicated to pushing the boundaries of scientific innovation. Currently, my passion lies in harnessing the power of CRISPR-Cas9 to bolster plant immunity against viruses, paving the way for a more resilient agricultural landscape. Besides my research, I’m deeply committed to addressing food insecurity and climate crisis. As a writer, I have published 4 books that demystify biotechnology for a broader audience, besides relevant articles and book chapters published in international journals. I firmly believe that gene editing holds the key to enhancing crop resilience and nutritional value, offering hope for a more equitable and sustainable food future.